Reducing human observation time and fatigue via automation.
Automated measurement devices reduce human error, reduce animal-human interactions, enhance measurement precision and saves time and resources.
Torrey Pines Instruments preclinical nociception and pruritus study products are exclusively licensed from the Anesthesiology Lab at UCSD, with demonstrated performance and efficacy. The UCSD Lab had supplied instruments in the past decade to over 300 institutional users and generated close on 1000 peer-reviewed publications using such instruments.
Industry Statistics
As of 2019 it is estimated that over 100 million animals are used every year by the research industry, which includes universities, pharmaceutical and diagnostic laboratories, as well as military and agricultural facilities. According to the United States Department of Agriculture’s (USDA) Animal and Plant Health Inspection Service (APHIS), the total number of animals used in research, testing and teaching in the U.S. in 2016 was 820,812. It is important to note that this number does not include mice, rats or birds. These animals, which are estimated to account for upwards of 95% of all animals used in science, are not required to be counted, as they do not fall under the protections of the Animal Welfare Act (AWA). The delta is a clear indice of how many mice and rats are used in research per year in the US.
Animals such as mice and rats are used in research in various ways, including as stand-ins for humans in biomedical, psychological and behavioral research.
Historically, the approach to measuring the stability and sources of variation within the nociceptive model of rat hind paw withdrawal from an induced pain event. The current model employment of human lab techs to observe and document the animal response, enables the inevitability of human error. Video capture and post processing of animal behavior is time and resource intensive.
The key limitation of the behavioral model is its labor-intensive nature and the time required to train observers.
Automated measurement devices reduce human error, reduce animal-human interactions, enhance measurement precision and saves time and resources.
Consistent, reliable and statistically sound data is the goal for every clinical study. Effective data management is essential to ensuring accurate data collection, entry, reports and validation, and has proven to be achieved by devices that are easy to use and intuitive for staff members. Clinical data integrity is always enhanced by the reduction of variability of data quality among individuals and teams involved.
In the field of medical research, a nociception assay evaluates the ability of a rodent to detect a harmful stimulus evoking pain, caused by stimulation of nociceptors. These assays measure the existence and level of pain through behaviors such as withdrawal, licking, immobility, and vocalization.
Torrey Pines Instruments’ goals are to enhance clinical data integrity and reduce the variability of data quality among individuals and teams involved in behavioral assay research.
Pain studies in humans are subjective, and are limited by ethical considerations, thus the common practice of testing on animals. Nociception, the ability to detect a potentially harmful stimulus, is a basic physiological function in animals but requires a particularly structured testing environment as animals cannot be said to be reporting pain.
In ANA, the injection of an irritant, such as formalin into the skin results in the persistent activation of small sensory afferents and will evoke an organized constellation of responses which includes favoring, licking and flinching of the injected paw.
The incidences of these nociceptive behaviors occur in two phases:
- Initial first phase (phase 1, 0-10 min)
- Delayed second phase (phase 2, 10-60 min).
The formalin test is a complex model that probes the pharmacology and physiology of systems that are activated by tissue injury.
The formalin test is a valid model for components of clinical pain associated with tissue injury. This places it in contrast to other models where the response is evoked by an acute, transient stimulus that does not produce tissue injury.
The biphasic display of paw-flinch behavior in the rat after injection of formalin into the dorsum of the hind paw is used for the screening of anti-hyperalgesic agents.
Characterized in ANA is a less labor-intensive system for counting flinch activity by detecting movement of a small metal band placed on the formalin-injected paw.
ANA Methodology
In ANA, a signal is generated as the band breaks the electromagnetic field of a loop antenna located under the rat and processed through an algorithm that determines flinch activity using:
- amplitude
- zero-voltage crossing
- signal duration
Flinches are summed and stored over a selected collection interval throughout the assay for later analysis. Studies have validated the measures with respect to:
- system stability over time
- system-to-“practiced observer” correlation on flinch detection, r2 = 0.94
- system variables including time of day, sex, age, and body weight
- 50% effective dose values similar to those previously reported for intrathecal morphine and the NMDA antagonist MK-801.
The escape response generated by a transient, strong stimulus attests to there being a close relationship between stimulus intensity, peripheral afferent discharge, and magnitude of the pain state as defined by response latency and magnitude.
There are situations, however, in which the magnitude of the response to pain may exceed what would normally be anticipated, given the magnitude of the physical stimulus and the afferent traffic generated by that stimulus.
These situations are loosely considered as reflecting a state of hyperalgesia, possibly arising from sensitization of the peripheral terminal and/or a central facilitation.
ANA has been developed to reflect the significance played by such facilitation on behavior. The common characteristic found in these models is the injury that is induced and its causing of the sensory axon to produce a persistent discharge.
ANA and the Formalin Test
A frequently used method of producing injury in the rat is the subcutaneous injection of a small volume of irritant such as formalin into its hind paw.
Typically, after the formalin injection, the rat displays a biphasic (phase I and phase II) incidence of flinching (rapid paw shaking) and licking of the injected paw. The behavioral syndrome produced by the injection of formalin into the paw has been widely used to define the pharmacology of systems that regulate facilitated processing.
The “formalin test” has evolved into a widely used tool in the screening of analgesic and anti-hyperalgesic drugs.
An important limitation of this behavioral model is its labor-intensive nature regarding data collection and the time required to train observers in its reliable implementation. To address this limitation, ANA assesses only the movement of the injected paw.
This approach involves placing a metal band on the injected paw and detecting the movement of that band with a localized low-strength sinusoidal electromagnetic field.
Description of the ANA System
The ANA device consists of a pair of electromagnetic coils: one serving as a transmitter and the other as receiver. When current passes through the transmitter coil, an electromagnetic field in the 6- to 8-kHz range is generated with a signal strength on the order of 5–8 mW.
Eddy currents are set up in ferrous or nonferrous metals within this field, and it is movement of the metal in the field that is detected.
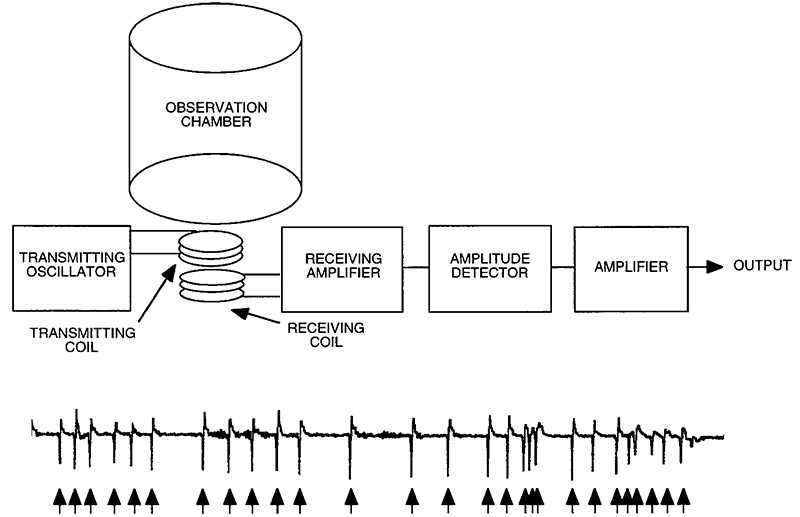
Location, size, electrical conductivity, and magnetic permeability are factors that determine how well movement of the metal is detected. The receiver output is amplified, filtered, and digitized for analysis (see Fig. 1).
The spatial displacement of the injected paw within the electromagnetic field is detected using a small metal collar placed on the paw.
During testing, the animal is placed into a cylindrical Plexiglas container (15 cm diameter × 30.5 cm height) mounted above the transmitter-receiver coil assembly, which is contained within a plastic enclosure.
The cylinder ensures that the rat will remain inside the electromagnetic field generated by the coil without the added stress of being in restraint.
ANA Paw-Band Parameters
In developing ANA, several parameters of the electromagnetic field and paw-band interaction were modeled to understand operating characteristics of the flinch detection system with the aim of defining the contributions of collar permeability, a complete or “O”-shaped collar configuration vs. a partial or “C”-shaped collar configuration, and relationship of collar mass and sinusoidal frequency.
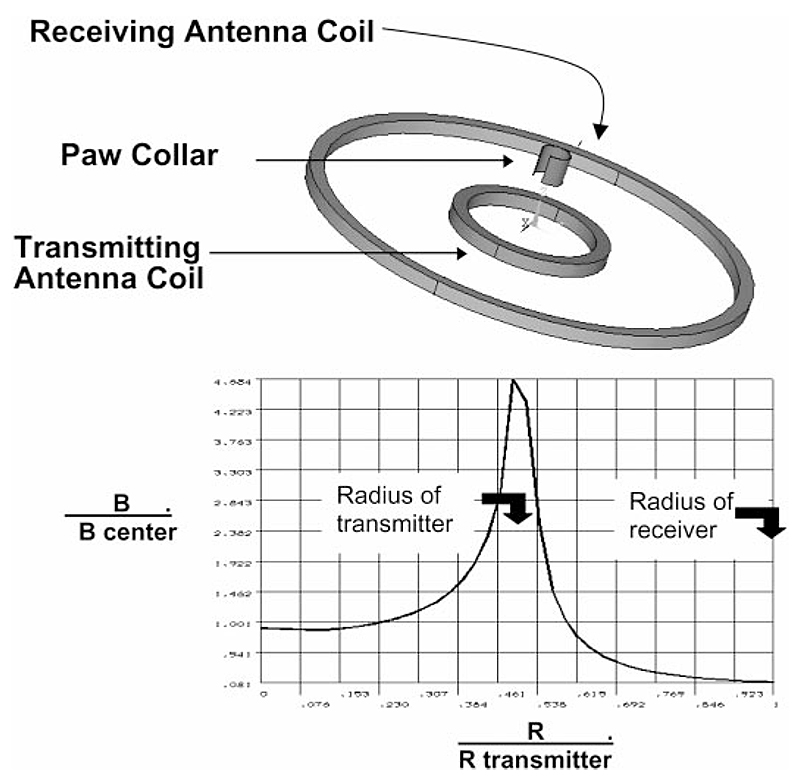
Bottom: graph displays the variation of the magnitude of the magnetic field when in phase with the transmitter current across the bore of the coils using a fixed-paw collar configuration. They-axis presents the dimensionless ratio of B (magnetic flux density at current location along the radius from coil center) divided by B center (magnetic flux density generated at the coil center). The x-axis shows the ratio of flux measured at the specified location along the radius (R) divided by the radius of the receiver (outer) coil (R transmitter).
The collar is centered with respect to the transmitter and receiver coils, thus simplifying the model to an axi-symmetrical representation of the collar and coils. The coil motion was limited to movement along the coil axis in a vertical direction (see Fig. 2).
Where the x-axis ratio is 1.0, the measurement is over the receiver coil. In the graph displayed, the transmitter coil is configured to be one-half of the radius of the receiver coil.
The collar is permeable, and even though it is changing its position, the motion of the collar to be treated as a steady-state condition. Whereas the direction of motion and coil dimensions affect the receiver output, the effects due to the collar material, collar thickness, and the open vs. intact configuration of the collar can be identified.
The relationship between sinusoidal current flow and generated EMF is linear; thus increasing energy will increase signal and system sensitivity.
ANA Data Collection
In ANA, signal events, which meet the criteria of a flinch, are captured and summed by time interval (normally set at 1-min increments). An example of a typical “real-time” signal showing flinch behavior is displayed in Fig. 3. Signals are often collected and stored in master raw data spreadsheets by animal according to its study and animal identifier. Software such as LabView or VisualBasic presents the identifiers and data for each animal, including animal numbers, treatment codes, dates, and other relevant information.
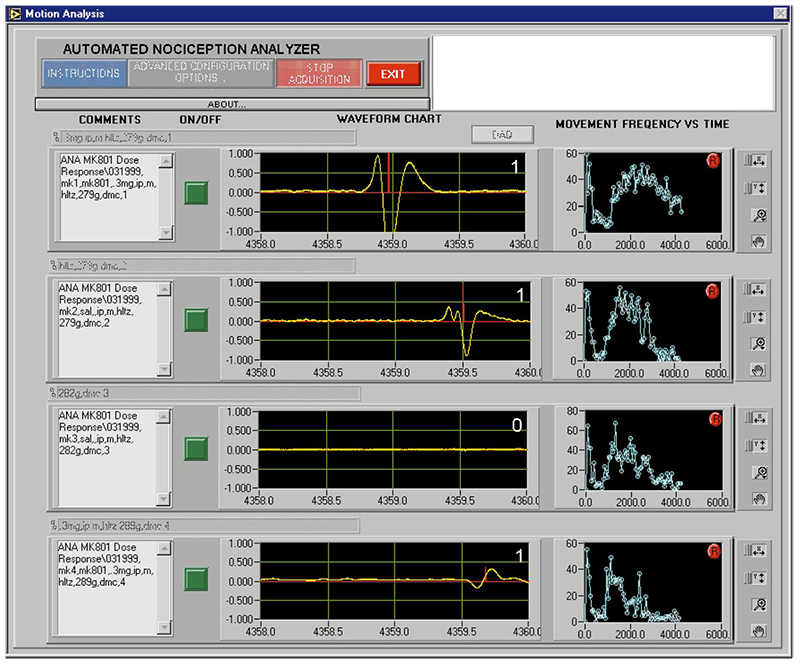
A window displaying the previous 2 seconds of digitized signal with markers indicating any flinch detection activity and a count of the flinches within that window. A line graph of each animal’s flinch count by sampling interval (1 min) since initiation of testing. A typical view of the computer screen during testing of four animals is shown in Fig. 3.
The view, for each animal, from left to right is1) study and animal; 2) small green virtual on/off switch that initiates and ceases data collection; 3) smoothed range waveform signal of previous 2 s with indicators for flinch activity and flinch count for that interval; and 4) minute-by-minute flinch count sums over completed portion of the study for each animal.
ANA – Common Preparation of Animal
A soft metal band (10 mm wide × 27 mm long, shaped into a C, and weighing ∼0.5 g) is placed on the hind paw of the animal being tested. The open part of the C is positioned at the top of the paw with the arms of the C gently compressed to form a bracelet around the paw (see Fig. 4. Retention of the band is enhanced by applying a small amount of adhesive (cyanoacrylate).
The size and weight of the band are sufficiently small so as not to hinder the animal’s normal movement. Animals are enabled to accommodate in individual Plexiglas chambers for 1 hour before being moved to a test chamber.
Just before the animal’s placement into the test chamber, it is briefly restrained in a cloth towel, and irritant (typically 5% formalin, in volumes of 50 μl) is injected into the dorsum of the banded paw. Data collection is initiated after the animal is placed inside the test chamber.
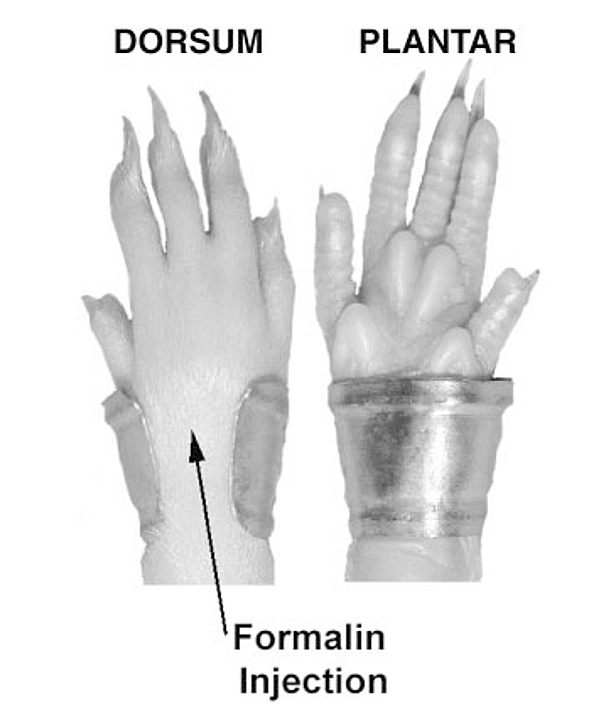
Each animal’s flinch count value over an interval of time (usually 1 min), forms the data set used in all subsequent analyses.
For graphic display of data, flinches per minute are normally presented, but the mean of the flinches observed over 5-min time periods (12 per hour) has been used to clarify the graph when a number of groups are being displayed for comparison of time effect.
For statistical comparison, the total number of flinches observed during any selected time period (phases I, II, IIA, and IIB) is calculated by accumulating each individual animal’s flinches over that time period and averaging the group.
Spreadsheet software used in conjunction with the data-collection software is constructed so as to allow the user to select up to four phases within the study’s time frame for making a phase-related analysis, including phase cumulative flinch averages, SDs, and SE values, and calculates percent maximum possible effect (%MPE), group means, SDs, and SE values when a group of control animals is available.
Typical ANA Time Courses
The flinch time course has frequently been divided into two principal phases: phase I (0–9 min) and phase II (10–60 min).
In this facility, it been observed that different drug effects may arise between early and late phase II; therefore, additional analyses occur at phase IIA (10–40 min) and phase IIB (41–60 min).
Dose-response slopes and values of effective dose in 50% of animals (ED50) with 95% confidence intervals are calculated by using a least squares linear regression analysis carried out on percent inhibition of the cumulative flinching number for each phase.
The percent inhibition is computed by dividing the response of each animal in a given group by the respective vehicle control group and multiplying by 100.
In studies, animals are often acclimated and then treated only with formalin injection just before the start of the 60-min data collection period.
For each phase, the following values are calculated:
- the Xbar chart center line value, which is an estimate of the process average and is computed by averaging subgroup averages;
- the Xbar chart control limits, which identify the distribution range within which the process can be considered in control and are computed as three times the estimate of the process SD divided by the square root of the subgroup size (n = 4);
- the S chart center line value, which is an estimate of the process variation computed by multiplying an unbiasing constant based on subgroup size (n = 4) by the process SD estimate;
- the S chart control limits, which identify the distribution range within which process variation can be considered in control and are computed as three times the estimate of the process SD times an unbiasing constant based on the subgroup size (n = 4);
- the percent upper and lower specification limits, which indicate the number of times the measured values exceeded either the upper or lower control limits and are displayed as a percentage of the total number of measured values; and
- the capability index (Cp), an indication of whether the process is in control and can stay within the limits specified as necessary for test relevance and calculated by dividing the upper and lower specification difference by six times the process SD estimate.
Upper and lower specification limits were calculated by phase as the mean of all tested animals ±3 × SD of the tested population, where, if the lower specification limit was <0, that limit became 0.
A Cp value >1.33 means that a very small number (6 out of 100,000) of the formalin tests do not fall within the limits considered relevant to the normal testing process (e.g., Cp < 1.0, process not capable; Cp = 1.0, process marginally capable; Cp > 1.0, process is capable).
Formalin-induced Behavioral Response
In ANA’s formation studies, the injection of formalin into the dorsum of one hind paw of 100 male Sprague-Dawley rats over a period of 10 mos showed a reliable biphasic flinching of the injected paw, with peak flinch rates during phases I and II being on the order of 50 and 37 flinches/min.

Figure 5 presents the time course of flinch responses, the mean cumulative flinches observed by phase [phase I: 203 ± 9 (SE); II: 1,058 ± 39; IIA: 792 ± 27; and IIB: 266 ± 16], and the statistical distribution of flinching in phases I and II. Analysis of this distribution (Kolmogorov-Smirnov) indicates that each phase was distributed normally (P> 0.9999).
Formalin Injection Effects
Injection of formalin into the paw, when utilized, leads to a biphasic flinching behavior with the magnitude of the behavior positively covaried with the concentration of formalin.
Injection of formalin induces a variety of complex, unconditioned behaviors, such as licking and guarding of the injected paw, which are reflective of a higher order motor organization.
Spinal transections markedly reduce phase I flexion extension of the formalin-injected hind paw, and phase II is abolished.
In addition to the paw withdrawal, formalin injection induces biphasic autonomic (cardiovascular) and supraspinally mediated hormonal responses.
Assessment of the firing patterns of dorsal horn wide dynamic range neurons, many of which are projection neurons, indicates that comparable formalin injections result in a similar biphasic activation pattern and an increase in the expression of c-Fos in dorsal horn neurons. Jointly, these phase II behaviors reflect augmented responses to spinofugal traffic and not simply an augmented reflex.
Mechanisms Underlying the Biphasic Components of the Flinching Behavior
Measurement of the firing pattern of small sensory afferents evoked by formalin injected into their receptive fields reveals an acute burst of activity that persists for several minutes. This initial discharge is followed by a persistent low level of afferent activity in slow (small diameter) and fast (large diameter) sensory afferents.
Temporally, the initial intense flinching (phase I; 0–10 min) correlates with the initial afferent barrage, whereas the prominent second phase of flinching (phase II; 20–60 min) corresponds with the interval when there is a relatively modest, but nonzero, level of afferent input.
Manual Formalin Testing
The evident utility of the formalin response indicates that it serves as a robust model for screening anti-hyperalgesic agents. A limitation is the time and training required to perform the test. In previously published work, the primary behavioral index has been the counting of the number of flinches in which 1-min flinching epochs are counted every 5 or 10 min over a 60-min observation interval. This spacing of observations permits a single technician to follow several rats concurrently. Other methods, such as the counting of time spent in multiple behaviors might provide additional sensitivity; however, the complexity of such an analysis places limitations on the implementation of the model.
First, it reduces the number of animals that can be followed concurrently.
Second, the need for observer vigilance means that, with repeated testing during the workday, the drift in operator reliability can surely be anticipated.
It is important to note that, in work published on the method, such practical issues as reliability among observers over time are not discussed.
Third, the use of indexes that involve judgments on weight bearing or appropriate licking behavior requires that the observer undergo extensive training and some form of technique validation to prove that needed skills have been acquired before it is considered that the data produced are useable.
Given the limitations associated with manual assessment of formalin-evoked flinching, efforts have been made to develop automated assessment systems. This was the premise of establishing ANA.
ANA’s Detector System
Radio-frequency interference and the interaction of the coil fields with the surrounding environment is minimized. Collar size is configured to be unencumbering to the rat and constructed of a metal with sufficient permittivity to generate a useful signal. The electromagnetic field and collar interaction has been undertaken to determine a workable system configuration, and a configuration has been defined that uses an open collar to produce a useful signal within a relatively localized field at the frequency (f) range of 6–8 kHz.
Computational analysis indicates that increased field strengths can be achieved by using higher coil voltage or by using a fixed signal strength and 1) metals of higher permittivity, 2) a higher sinusoidal f, and/or 3) increasing field density by decreasing the field coil size.
ANA and the Automated Formalin System
The methods utilized in ANA to determine system relevance obtained human and system flinch counts concurrently and compared flinch-count data obtained from previous drug studies with similar new studies using the automated system.
Concurrent paw-flinch assessment by a trained human observer and by the automated system demonstrated good correlation. There is a tendency for the automatic flinch counts to be higher than those obtained by the observer, but this is believed to reflect the inability of the human observer to follow the rapid flinching that is evident during that phase.
With regard to the activity of drugs known to alter flinching behavior, morphine given intrathecally and systemically produced a dose-dependent reduction in all phases of the response to formalin.
These results are consistent with the mechanism of action of morphine, in which, at the spinal level, μ-opiate receptors are believed to diminish the excitability of input associated with the activation of small, primary afferents. The ED50 values obtained from the automated assessment correspond closely with the ED50 values reported using human scoring. Intrathecal delivery of an NMDA-receptor blocker, MK-801, has been shown to block phase II of the response to formalin and, to a lesser degree, phase I in a dose-dependent manner.
Assessment of System Reliability
In any assay system, baseline stability over time is a primary concern. In ANA studies, baseline flinching behavior is assessed in separate studies over 5-day, 7-wk, and 11-mo time increments. Over these intervals, there are no statistically significant shifts in baseline activity in standard, untreated animals.
A more definite approach is to undertake a statistical process analysis. Using statistical process control analysis over a particular time frame, increment, the process, measurement of paw flinches, was in control (i.e., paw-flinch measurement varied only within the limits of a selected statistical distribution) and capable (i.e., a high percentage of the measurements taken fell within previously determined specification limits).
A process that is in control, or stable, can be defined by a normal distribution of the observations obtained over time. One measure of process control is the Cp. This measure defines how satisfactory a process is at meeting the requirements placed on it. A Cp value of >1.00 means that only a small number of the formalin tests would not fall within the limits considered relevant to the normal testing process. Conversely, a Cp < 1.0 indicates that a significant number of observations fell outside of specified distribution. In the present studies, Cp values for both phases I and II over a 7-wk interval were >1.000 and thus in control and capable.
Although seldom used in behavioral system studies, these types of analyses can 1) bolster confidence in a testing process, 2) help to define its sensitivity limits, and 3) aid in the detection of transient or systematic changes before they affect study results.
Variables Influence the Formalin Response
Several variables may impact the magnitude of the flinching behavior. With the use of the automated assessment systems, selected variables are examined.
Behavioral indexes.
Injection of formalin into the paw results in a variety of spontaneous behaviors including flinching of the paw, licking of the paw, lifting of the paw from the surface of the chamber, changes in weight bearing, and vocalization.
Assessment of the intensity of a behavior induced by formalin injection has typically involved one or a combination of measures, including the measurement of duration and/or f for one or more of the known behaviors In most cases, as formalin concentration increases or as the dose of an analgesic agent (e.g., morphine) decreases.
Weighted measures (e.g., time of licking and flicking) may provide a more robust index with a greater dynamic response at lower formalin concentrations than other simple indexes such as flinching. Choice of indexes may be influenced by the time required to complete a test and the level of training required to achieve reliability.
Concentration of formalin.
Increasing formalin concentration, typically over a range of 0.5 to 5%, leads to a more intense and progressively longer lasting flinch response. Assessment of the intensity of several pain behaviors has shown that, as formalin concentration rises, the magnitude or incidence of the measured behaviors, blood pressure, or flinching increases. In present ANA studies, a plateau effect is observed such that the maximum was observed at 2.5%. These results, obtained with automated flinch counting, are in accord with previous work using weighted behavioral measures and blood pressure.
Overall Benefits of ANA
ANA represents the development of an automated sensing system that counts the spatial displacement of the injected hind paw. This system has been shown to describe a response count and distribution that resembles that obtained with manual counting systems.
The formalin test reflects a neural substrate that involves the generation and support of a facilitated state of processing. Behavior observed during phase II appears to arise out of the initial barrage of afferent traffic and continuous low-level input found in a formalin-induced injury.
The response of this system is such that it appears to reveal a pharmacology that is considered to reflect facilitated states of processing.
The strength of ANA lies in the ability to screen large numbers
of animals on a daily basis, absence of operator fatigue, a likely increase in
reliability over repeated tests, and reduced training time.